Resisted sled sprint training (RSST) is a common training method used to enhance sprint performance in athletes (2,38). Despite the mechanical similarity between RSST and unresisted sprinting, it has been hypothesised that the decrease in running velocity and changes in sprinting kinematics caused by RSST may limit the transfer of training of RSST to sprinting (2,3,26). The purpose of this article is to review the literature on RSST focussing solely on the sled towing exercise, discuss its effectiveness as a mean to enhance short sprint performance, defined in this article by sprints up to 20m, and provide practical recommendations for the implementation of RSST.
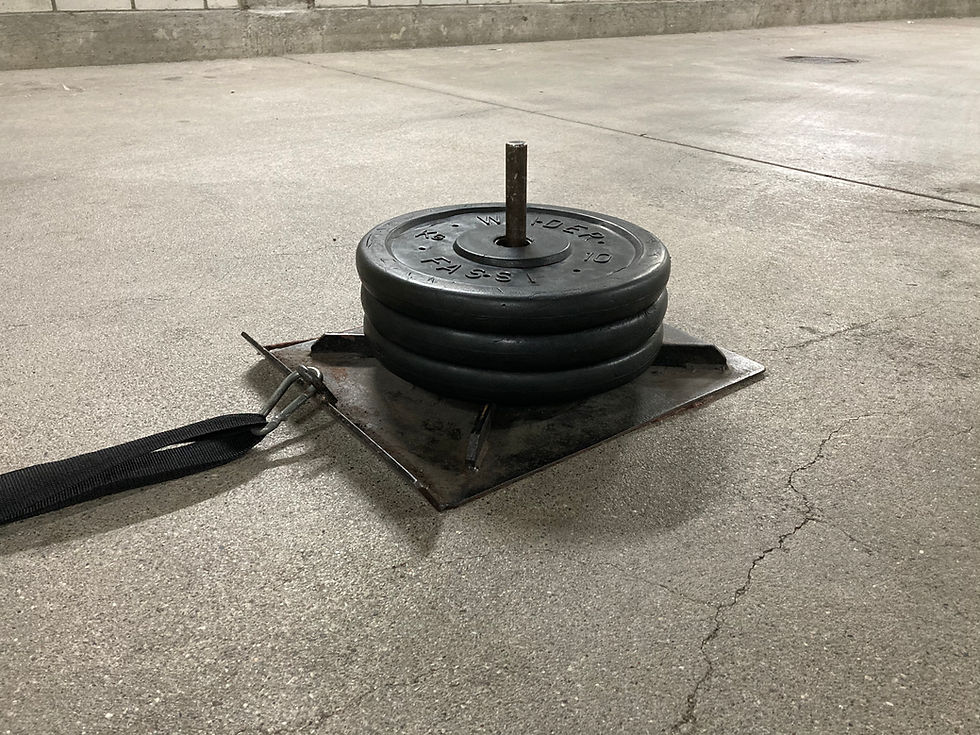
Key Determinants of Sprinting
Short sprint performance is associated with performance in numerous sports (21). Running speed is therefore a fundamental target in the training of athletes, especially in court- and field-based sports, and the determinants of sprint performance have been studied to offer insight on the qualities displayed by faster runners. An increase in lower body strength has been shown to transfer positively to sprint performance, particularly in sprint distances below 30m (42). Force application technique, specifically the effectiveness of horizontal force application, has also been found to determine sprint performance (33,39). Combining sprinting kinetics and kinematics, propulsive impulse explains most of the variance in short sprint performance (8,16,19). The magnitude and the effectiveness of force output may therefore be considered the two key determinants of sprint performance.
Rationale for Resisted Sled Sprint Training
Improvements in sprint performance are typically targeted with technical training, resistance training, plyometric training, as well as assisted and resisted sprint training (11,41). Unresisted and resisted sprint training have been shown to result in greater improvements than other methods in 10 and 20-m sprint performance (41). Despite lower force production compared to half squats, jump squats and unloaded countermovement jumps, RSST provides greater specificity with consideration of movement velocity and coordination (36). This review will focus on the transfer of RSST to short sprint performance by considering the impact of RSST on the two key determinants of sprint performance, the magnitude and the effectiveness of force production, excluding the post activation potentiation effect that RSST may have.
Sprinting Kinematics
Sprinting is acutely affected in RSST with a decrease in stride length, stride frequency and flight time, and an increase in ground contact time (GCT), associated with an increase in trunk lean throughout the stance phase and greater hip, knee and ankle flexion at foot strike (5,11,17,26,30,31,35). These changes in kinematics typically occur with loads greater than 20% body mass (%BM), while loads that cause less than 10% decrement in velocity (%Vdec) cause minimal changes (1,11,22,26,28,30,31). Attaching the harness at the waist also causes less kinematic alterations compared with an attachment at the shoulders (5). It was initially recommended to avoid these changes and until 2014 research was mostly performed with loads that caused a maximum 10%Vdec. However, it is useful to consider the impact of the kinematic changes during RSST. As a result of a greater forward lean of the body, an anterior shift of the athlete’s centre of mass (COM) allows a more posterior foot strike under the COM (11,22,46). With a decreased touchdown distance, braking forces are reduced and propulsion during GCT is optimised (20). The slower sprint times in RSST are mainly explained by reduced stride length rather than stride frequency (11,26,35). Whether these changes remain in sprinting after RSST has not been established unanimously. An increased trunk lean has been observed after RSST (1,43). Kawamori et al. (18) reported an increased initial step length and stride frequency in early acceleration. However, other studies reported no change in stride length and stride frequency (1,22,43) or only an increase in stride rate (46). As a greater trunk lean is associated with an acceleration pattern (10,26), the kinematic adaptations resulting from RSST may therefore provide athletes with a more efficient motor solution for short sprint performance.
Sprinting Kinetics
RSST is characterised by greater GCT and propulsive phase duration than in unresisted sprint training (UST) (5) and increasing loads result in a further increase in GCT (26). A decrease in braking ground reaction force (GRF) results from the kinematic adaptations discussed above (17). This decrease is greater with heavier loads (17,37). Compared with UST, athletes produce greater propulsive and horizontal impulse and mean force during RSST (5). The magnitude of this increase appears to depend on the load used. RSST with 10%BM does not appear to be sufficient to affect propulsive GRF and acceleration (9,17,29) which are improved with 20-30%BM (9,17). A greater peak rate of force development also occurs at 20%BM, compared with 10 and 15%BM (29). The greater production of horizontal GRF causes an increase in the ratio of forces (RF), which means that more horizontal force is produced relative to vertical GRF (17). Greater horizontal impulse is also produced with a harness attachment at the waist than at the shoulders (5). Finally, the strength level of the athletes and the load used affect power output. Stronger athletes produce greater maximal power (Pmax) at greater velocities and Pmax occurs at 69-96%BM at 50%Vdec (12,13).
Adaptations to RSST
Improvements in short sprint performance have been observed following RSST (2,7,22,34,38). However, in the early research that considered almost exclusively loads up to 10%Vdec, UST was considered as effective as RSST (2,38). It is therefore useful to review the specific adaptations to RSST. Generally, greater improvements occur with loads of 40-133%BM that cause 30-75%Vdec (7,18,34,40). Luteberget et al. (27) reported small changes in muscle architecture after RSST with 12.4%BM, with a decreased pennation angle (2.8%) and increased fascicle length (4%) in the vastus lateralis. Interestingly, these changes had an almost perfect correlation with the change in 10-m time. However, greater changes occurred in UST (6% and 5.3%), and better physiological adaptations as well as increased lower limb extension force can be achieved with methods such as traditional strength training and plyometric training (41).
The main adaptations to RSST are changes in the sprinting force-velocity (FV) profile, specifically an increase in Pmax(7,22,32), an increase in maximal theoretical horizontal force (F0) (7,22,32,34) and a decrease in RF (22,34). Athletes that have greater initial horizontal force production capability benefit from a greater improvement in Pmax (14,23). The impact on the FV profile is also load-specific (7). Overall, adaptations are optimised at 50%Vdec, since a decreased in theoretical maximal velocity is minimised (7). The main adaptations to RSST may thus be summarised as greater horizontal force application capability and better mechanical effectiveness (34).
Practical Applications
Adaptations to RSST are specific to distance (41), resistance (7,22), training volume (2), and to the athletes’ initial FV profile (14,23). Programming of RSST should therefore have a specific target.
Different methods have been used to prescribe resistance: load (kg), %BM, %Vdec, and maximal resisted sled load (MRSL), which is the maximal load that allows the athlete to maintain acceleration for 20m (28). Since force of static friction accounts for much of the resistance in short sprints (11) and friction depends on various factors such as ground surface (24), it is recommended to use %Vdec or %MRSL. For contact sports such as rugby, where momentum (mass x velocity) is important pre- and post-contact and may be considered a desired adaptation, Tierney et al. (44) suggested the use of maximal system (athlete + resistance) momentum to optimise loading and the transfer to unresisted sprint momentum.
An initial FV profiling may be useful to prescribe targeted training strategies (22). As a general guideline, Cahill et al. (6) have proposed to individualise loading using four %Vdec zones based on training focus: <10% high speed, <35% speed-strength, 50% power, >65% strength-speed. Heavier loads provide adaptations that are specifically effective for initial acceleration and short sprint performance. It is therefore recommended to use resistance in the power and strength-speed zones for distances between 5-20m, 2-3x/week for at least 4-6 weeks (2,38). For a better transfer, it is also recommended to combine RSST with UST (45). A 6-week biweekly pre-season example of RSST for well-trained athletes is provided in Table 1. Peak adaptations occur 2-4 weeks after a RSST block (32). A tapering phase is therefore suggested post-RSST when aiming for optimised adaptations.
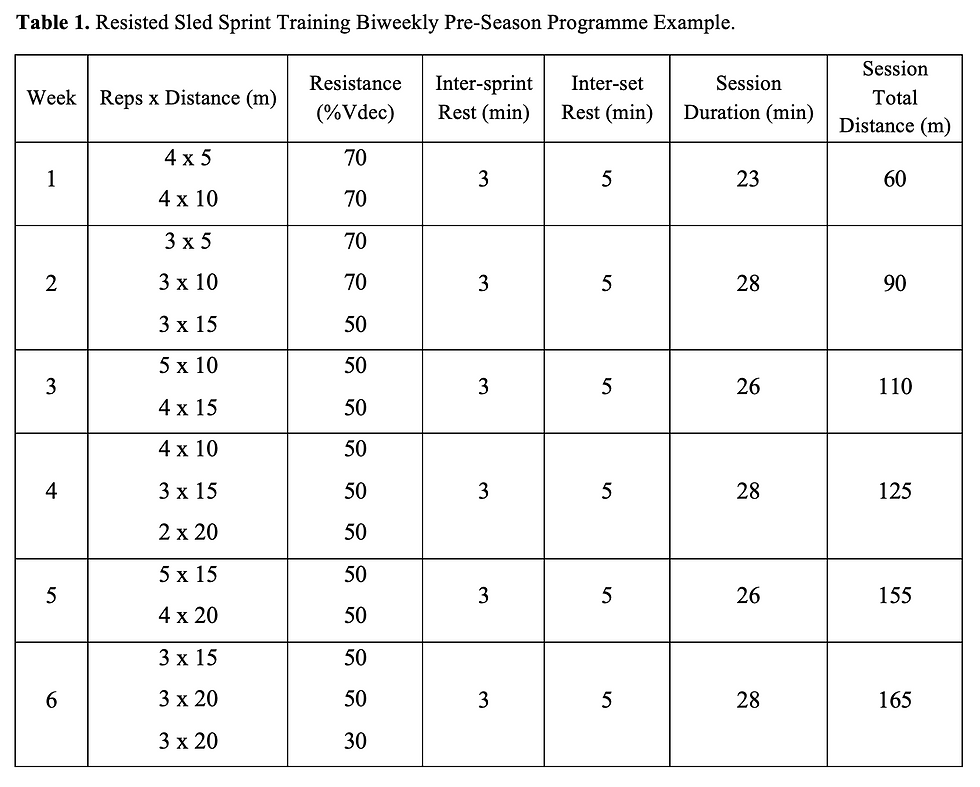
It should be noted that RSST causes metabolic stress with an acute increase in blood lactate, particularly with greater resistance (4). Creatine kinase is still elevated after 24h but short sprint performance is not affected (4). Greater resistance also requires longer within-session rest (4), with a greater impact on weaker and less powerful athletes (25). A minimum of 3-min inter-sprint rest is therefore suggested with minimum 48h between sessions.
Conclusion
RSST is an effective method to improve short sprint performance. With positive adaptations in power output capacity and in skill through an increase in horizontal force production and mechanical effectiveness, it improves mainly the F0, Pmax, and RF components of the FV profile. To optimise adaptations, the load should be prescribed based on athletes’ FV profile, which has been shown to be more individual than sport-specific (15). Without a FV profile, a Pmax resistance that leads to 50%Vdec may be used to target adaptations in sprint-specific power. Finally, RSST should be implemented in combination with UST before improvements in short sprint performance may be optimised.
References
1. Alcaraz, P. E., Elvira, J. L. L., & Palao, J. M. (2014). Kinematic, strength, and stiffness adaptations after a short-term sled towing training in athletes: Short-term sled towing training in athletes. Scandinavian Journal of Medicine & Science in Sports, 24(2), 279–290.
2. Alcaraz, P. E., Carlos-Vivas, J., Oponjuru, B. O., & Martínez-Rodríguez, A. (2018). The effectiveness of resisted sled training (RST) for sprint performance: A systematic review and meta-analysis. Sports Medicine, 48(9), 2143–2165.
3. Alcaraz, P. E., Palao, J. M., & Elvira, J. L. L. (2009). Determining the optimal load for resisted sprint training with sled towing. Journal of Strength and Conditioning Research, 23(2), 480–485.
4. Bachero-Mena, B., Sánchez-Moreno, M., Pareja-Blanco, F., & Sañudo, B. (2020). Acute and short-term response to different loading conditions during resisted sprint training. International Journal of Sports Physiology and Performance, 15(7), 1–8.
5. Bentley, I., Atkins, S. J., Edmundson, C. J., Metcalfe, J., & Sinclair, J. K. (2016). Impact of harness attachment point on kinetics and kinematics during sled towing. Journal of Strength and Conditioning Research, 30(3), 768–776.
6. Cahill, M. J., Cronin, J. B., Oliver, J. L., Clark, K. P., Lloyd, R. S., & Cross, M. R. (2019). Sled pushing and pulling to enhance speed capability. Strength and Conditioning Journal, 41(4), 94–104.
7. Cahill, M. J., Oliver, J. L., Cronin, J. B., Clark, K., Cross, M. R., Lloyd, R. S., & Lee, J. E. (2020). Influence of resisted sled-pull training on the sprint force-velocity profile of male high-school athletes. Journal of Strength and Conditioning Research, 34(10), 2751–2759.
8. Colyer, S. L., Nagahara, R., & Salo, A. I. T. (2018). Kinetic demands of sprinting shift across the acceleration phase: Novel analysis of entire force waveforms. Scandinavian Journal of Medicine & Science in Sports, 28(7), 1784–1792.
9. Cottle, C. A., Carlson, L. A., & Lawrence, M. A. (2014). Effects of sled towing on sprint starts. Journal of Strength and Conditioning Research, 28(5), 1241–1245.
10. Cronin, J., & Hansen, K. T. (2009). Resisted sprint training for the acceleration phase of sprinting. Strength and Conditioning Journal, 28(4), 42–51.
11. Cronin, J., Hansen, K., Kawamori, N., & McNair, P. (2008). Effects of weighted vests and sled towing on sprint kinematics. Sports Biomechanics, 7(2), 160–172.
12. Cross, M. R., Brughelli, M., Samozino, P., Brown, S. R., & Morin, J.-B. (2017). Optimal loading for maximizing power during sled-resisted sprinting. International Journal of Sports Physiology and Performance, 12(8), 1069–1077.
13. Cross, M. R., Samozino, P., Brown, S. R., & Morin, J.-B. (2018). A comparison between the force–velocity relationships of unloaded and sled-resisted sprinting: Single vs. multiple trial methods. European Journal of Applied Physiology, 118(3), 563–571.
14. Escobar Álvarez, J. A., Jiménez Reyes, P., Da Conceição, F. A., & Fuentes García, J. P. (2020). Does the initial level of horizontal force determine the magnitude of improvement in acceleration performance in rugby? European Journal of Sport Science, 1–9. https://doi.org/10.1080/17461391.2020.1793004
15. Haugen, T. A., Breitschädel, F., & Seiler, S. (2019). Sprint mechanical variables in elite athletes: Are force-velocity profiles sport specific or individual? PLOS ONE, 14(7), e0215551.
16. Hunter, J. P., Marshall, R. N., & McNair, P. J. (2005). Relationships between ground reaction force impulse and kinematics of sprint-running acceleration. Journal of Applied Biomechanics, 21(1), 31–43.
17. Kawamori, N., Newton, R., & Nosaka, K. (2014). Effects of weighted sled towing on ground reaction force during the acceleration phase of sprint running. Journal of Sports Sciences, 32(12), 1139–1145.
18. Kawamori, N., Newton, R. U., Hori, N., & Nosaka, K. (2014). Effects of weighted sled towing with heavy versus light load on sprint acceleration ability. Journal of Strength and Conditioning Research, 28(10), 2738–2745.
19. Kawamori, N., Nosaka, K., & Newton, R. U. (2013). Relationships between ground reaction impulse and sprint acceleration performance in team sport athletes. Journal of Strength and Conditioning Research, 27(3), 568–573.
20. Kugler, F., & Janshen, L. (2010). Body position determines propulsive forces in accelerated running. Journal of Biomechanics, 43(2), 343–348.
21. Laakso, L. A. (2020). Critical evaluation of the physiological adaptations to repeated-sprint training: Implications for training recommendations and repeated-sprint ability of well-trained field-based team-sport athletes. Journal of Australian Strength and Conditioning, 28(4), 75–81.
22. Lahti, J., Huuhka, T., Romero, V., Bezodis, I., Morin, J.-B., & Häkkinen, K. (2020). Changes in sprint performance and sagittal plane kinematics after heavy resisted sprint training in professional soccer players. PeerJ, 8, e10507.
23. Lahti, J., Jiménez-Reyes, P., Cross, M. R., Samozino, P., Chassaing, P., Simond-Cote, B., Ahtiainen, J. P., & Morin, J.-B. (2020). Individual sprint force-velocity profile adaptations to in-season assisted and resisted velocity-based training in professional rugby. Sports, 8(5), 74.
24. Linthorne, N. P., & Cooper, J. E. (2013). Effect of the coefficient of friction of a running surface on sprint time in a sled-towing exercise. Sports Biomechanics, 12(2), 175–185.
25. Lizana, J. A., Bachero-Mena, B., Calvo-Lluch, A., Sánchez-Moreno, M., Pereira, L. A., Loturco, I., & Pareja-Blanco, F. (2020). Do faster, stronger, and more powerful athletes perform better in resisted sprints? Journal of Strength and Conditioning Research. Advance online publication. https://doi.org/10.1519/JSC.0000000000003719
26. Lockie, R. G., Murphy, A. J., & Spinks, C. D. (2003). Effects of resisted sled towing on sprint kinematics in field-sport athletes. Journal of Strength and Conditioning Research, 17(4), 760–767.
27. Luteberget, L. S., Raastad, T., Seynnes, O., & Spencer, M. (2015). Effect of traditional and resisted sprint training in highly trained female team handball players. International Journal of Sports Physiology and Performance, 10(5), 642–647.
28. Martínez-Valencia, M. A., González-Ravé, J. M., Santos-García, D. J., Alcaraz Ramón, P. E., & Navarro-Valdivielso, F. (2014). Interrelationships between different loads in resisted sprints, half-squat 1 RM and kinematic variables in trained athletes. European Journal of Sport Science, 14(sup1), S18–S24.
29. Martínez-Valencia, M. A., Romero-Arenas, S., Elvira, J. L. L., González-Ravé, J. M., Navarro-Valdivielso, F., & Alcaraz, P. E. (2015). Effects of sled towing on peak force, the rate of force development and sprint performance during the acceleration phase. Journal of Human Kinetics, 46(1), 139–148.
30. Maulder, P. S., Bradshaw, E. J., & Keogh, J. W. (2008). Kinematic alterations due to different loading schemes in early acceleration sprint performance from starting blocks. Journal of Strength and Conditioning Research, 22(6), 1992–2002.
31. Monte, A., Nardello, F., & Zamparo, P. (2017). Sled towing: The optimal overload for peak power production. International Journal of Sports Physiology and Performance, 12(8), 1052–1058.
32. Morin, J.-B., Capelo-Ramirez, F., Rodriguez-Pérez, M. A., Cross, M. R., & Jimenez-Reyes, P. (2020). Individual adaptation kinetics following heavy resisted sprint training. Journal of Strength and Conditioning Research. Advance online publication. https://doi.org/10.1519/JSC.0000000000003546
33. Morin, J.-B., Edouard, P., & Samozino, P. (2011). Technical ability of force application as a determinant factor of sprint performance. Medicine and Science in Sports and Exercise, 43(9), 1680–1688.
34. Morin, J.-B., Petrakos, G., Jiménez-Reyes, P., Brown, S. R., Samozino, P., & Cross, M. R. (2017). Very-heavy sled training for improving horizontal-force output in soccer players. International Journal of Sports Physiology and Performance, 12(6), 840–844.
35. Murray, A., Aitchison, T., Ross, G., Sutherland, K., Watt, I., McLean, D., & Grant, S. (2005). The effect of towing a range of relative resistances on sprint performance. Journal of Sports Sciences, 23(9), 927–935.
36. Okkonen, O., & Häkkinen, K. (2013). Biomechanical comparison between sprint start, sled pulling, and selected squat-type exercises. Journal of Strength and Conditioning Research, 27(10), 2662–2673.
37. Pantoja, P. D., Carvalho, A. R., Ribas, L. R., & Peyré-Tartaruga, L. A. (2018). Effect of weighted sled towing on sprinting effectiveness, power and force-velocity relationship. PLOS ONE, 13(10), e0204473.
38. Petrakos, G., Morin, J.-B., & Egan, B. (2016). Resisted sled sprint training to improve sprint performance: A systematic review. Sports Medicine, 46(3), 381–400.
39. Rabita, G., Dorel, S., Slawinski, J., Sàez‐de‐Villarreal, E., Couturier, A., Samozino, P., & Morin, J.-B. (2015). Sprint mechanics in world-class athletes: A new insight into the limits of human locomotion. Scandinavian Journal of Medicine and Science in Sports, 25(5), 583–594.
40. Rodríguez-Rosell, D., Sáez de Villareal, E., Mora-Custodio, R., Asián-Clemente, J. A., Bachero-Mena, B., Loturco, I., & Pareja-Blanco, F. (2020). Effects of different loading conditions during resisted sprint training on sprint performance. Journal of Strength and Conditioning Research. Advance online publication. https://doi.org/10.1519/JSC.0000000000003898
41. Rumpf, M. C., Lockie, R. G., Cronin, J. B., & Jalilvand, F. (2016). Effect of different sprint training methods on sprint performance over various distances: A brief review. Journal of Strength and Conditioning Research, 30(6), 1767–1785.
42. Seitz, L. B., Reyes, A., Tran, T. T., Sáez de Villarreal, E., & Haff, G. G. (2014). Increases in lower-body strength transfer positively to sprint performance: A systematic review with meta-analysis. Sports Medicine, 44(12), 1693–1702.
43. Spinks, C. D., Murphy, A. J., Spinks, W. L., & Lockie, R. G. (2007). The effects of resisted sprint training on acceleration performance and kinematics in soccer, rugby union, and Australian Football players. Journal of Strength and Conditioning, 21(1), 77–85.
44. Tierney, P., Reardon, C., & Delahunt, E. (2019). Momentum: A practical solution to calculate the optimal load for resisted sled sprint training. Sport Performance and Science Reports, 68.
45. West, D. J., Cunningham, D. J., Bracken, R. M., Bevan, H. R., Crewther, B. T., Cook, C. J., & Kilduff, L. P. (2013). Effects of resisted sprint training on acceleration in professional rugby union players. Journal of Strength and Conditioning Research, 27(4), 1014–1018.
46. Zafeiridis, A., Saraslanidis, P., Manou, V., Ioakimidis, P., Dipla, K., & Kellis, S. (2005). The effects of resisted sled-pulling sprint training on acceleration and maximum speed performance. Journal of Sports Medicine and Physical Fitness, 45(3), 284–290.
Comments